When assessing salt curves, testing is commonly performed using a simple viscometer. These viscometers provide limited data, as they don’t have the ability to measure viscoelasticity, or viscosity as a function of shear rate. This limits the ability to measure the shear-dependent viscosity of non-Newtonian fluids. Utilising our rotational rheometers, we can uncover a whole world of new insights into formulations.
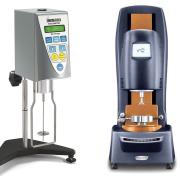
Our rotational rheometers can measure viscosity at different shear rates, predicting the behaviour of the formulations at various stages, such as resting in the bottle, dispensing, and application into the hands and hair. Smooth dispensing, required stability, and intended user experience depend on optimal viscosity. Viscoelasticity measurements can provide further insights into lubricity and resistance to extension, providing valuable insights into how the product performs under conditions that mimic actual use, which is discussed further in this case study.
Salt is a crucial ingredient in formulations, especially when it comes to influencing the viscosity and viscoelasticity of products like shampoos. Formulating shampoos, soaps, and body washes requires an understanding of the “Salt Curve” phenomenon, which describes the range of salt concentrations wherein a surfactant solution undergoes changes in its viscosity. Adding salt to surfactants initially increases viscosity, but excessive salt diminishes viscosity.
Salty Science – The Role of Salt in Micelle Formation
Many formulations contain surfactants, from detergents and emulsifiers to shampoos and creams. Surfactants are molecules that lower surface tension and adsorb at interfaces. They are amphiphiles, meaning they have a two-part structure: one part has little attraction for water (hydrophobic), whilst the other part has a strong attraction for water (hydrophilic). Once all the interfaces are saturated, surfactants aggregate into micelles with different geometries forming an “electrical double-layer”. An example of a surfactant molecule and a spherical anionic micelle structure can be seen below in Figure 2 and 3.
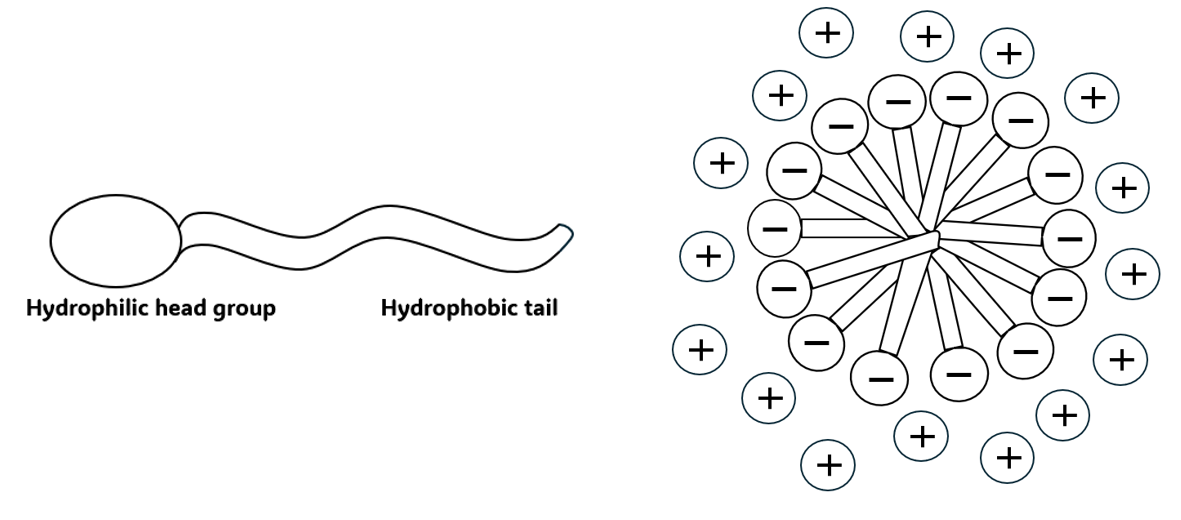
When salt is added to solution, it interacts with the heads of the surfactant molecules, causing them to pack closer together. This results in stronger interactions between surfactants and a denser micelle network, which results in an increase in viscosity. However, when too much salt is added, the viscosity starts to decrease resulting in oversalting. This is due to the electrical double layer becoming compressed, leading to an increase in the attractive forces between micelles, causing them to aggregate and form larger micelle geometries.
Exploring Salt Curves: Our Methodology
To investigate the effect of salt addition on the viscosity and viscoelasticity, surfactant solutions were made to final concentrations of 26%
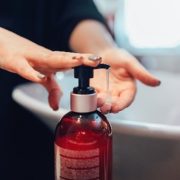
sodium laureth sulfate (SLES), 6% cocamidopropyl betaine (CAPB), and 68% deionised water. The solution was divided into 10 equal-weight samples. Sodium chloride salt was then added to each of these samples to give a range of salt concentrations from 0.2% to 2% in 0.2% increments. Samples were mixed until homogenous and left to stand before testing. Testing was performed on a rheometer to measure the viscosity and viscoelasticity (normal stress) as a function of shear rate. A solvent trap cover was employed to minimise drying of the samples at the exposed edge.
Impact of Salt on Viscosity
The perceived value of a shampoo can be correlated to its viscosity. A highly viscous shampoo can create a “rich” feel; however, it may be more difficult to handle and use. In contrast, a shampoo with a low viscosity may be perceived as “watered down” and less valuable. Different viscosity criteria apply depending on the intended application and target consumer. For example, a children’s shampoo is required to be gentle to use, which necessitates a reduced viscosity. This underscores the importance of tailoring the viscosity to the formulation’s specific requirements.
In addition to this, many luxury shampoos contain pearlescent pigments to give a creamy appearance. It is imperative to maintain these pigments in suspension such that they provide the desired effect to the consumer over the intended shelf life. Viscosity data collected can be correlated to the stability of these pigments within suspension, which can be extremely valuable for formulators.
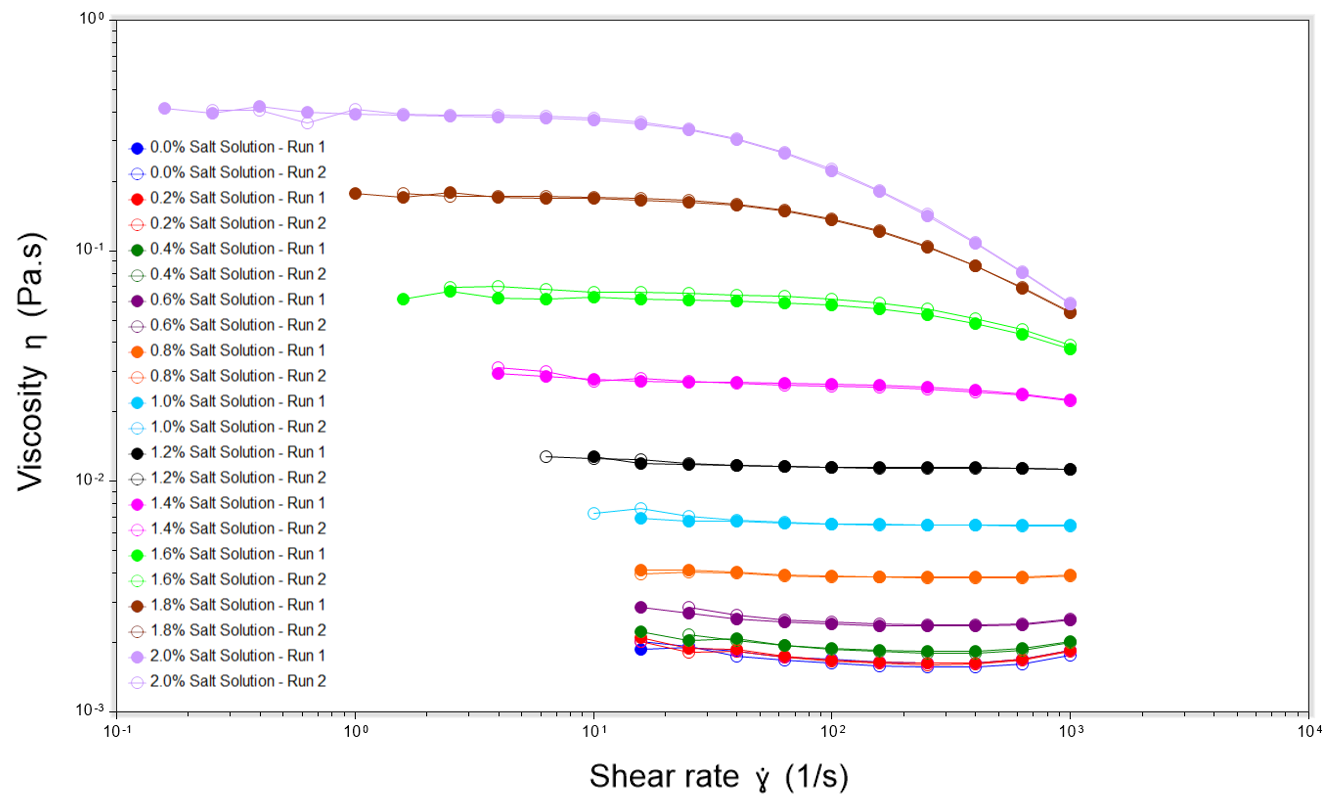
The results show that increasing salt in a surfactant solution results in a higher viscosity, with a change in viscosity over two decades observed. Over our sample range, a decrease in viscosity from the addition of salt was not observed. It can be expected that if testing was performed with higher concentrations of salt within solution, a decrease in viscosity would eventually be observed.
Solutions containing salt concentrations between 0% and 1.2% exhibit apparent Newtonian behaviour, with viscosity remaining relatively constant across the range of shear rates, close to the zero-shear viscosity. When the salt concentration is increased to 1.4% and above, the samples begin to exhibit non-Newtonian shear-thinning behaviour at higher shear rates. This behaviour is particularly beneficial for shampoos with a high zero shear viscosity, as it allows for easier dispensing from the bottle, while still providing the luxury feel when the product is experiencing low shear rates in the consumer’s hands.
Impact of Salt on Viscoelasticity
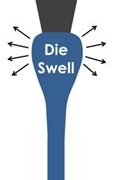
Viscoelasticity can be understood through normal stress measurements. In the context of shampoos, these measurements provide valuable insights into how the product behaves under conditions that mimic actual use, such as when the shampoo is dispensed or spread on hair.
Higher normal stress typically correlates with resistance to extension, manifesting behaviours such as tailing when dispensing, or die swell when extruding. This can be related to the extensional viscosity, which helps the product maintain a fluid film during squeezing, contributing to lubricity and hence affecting the luxurious feel of a shampoo.
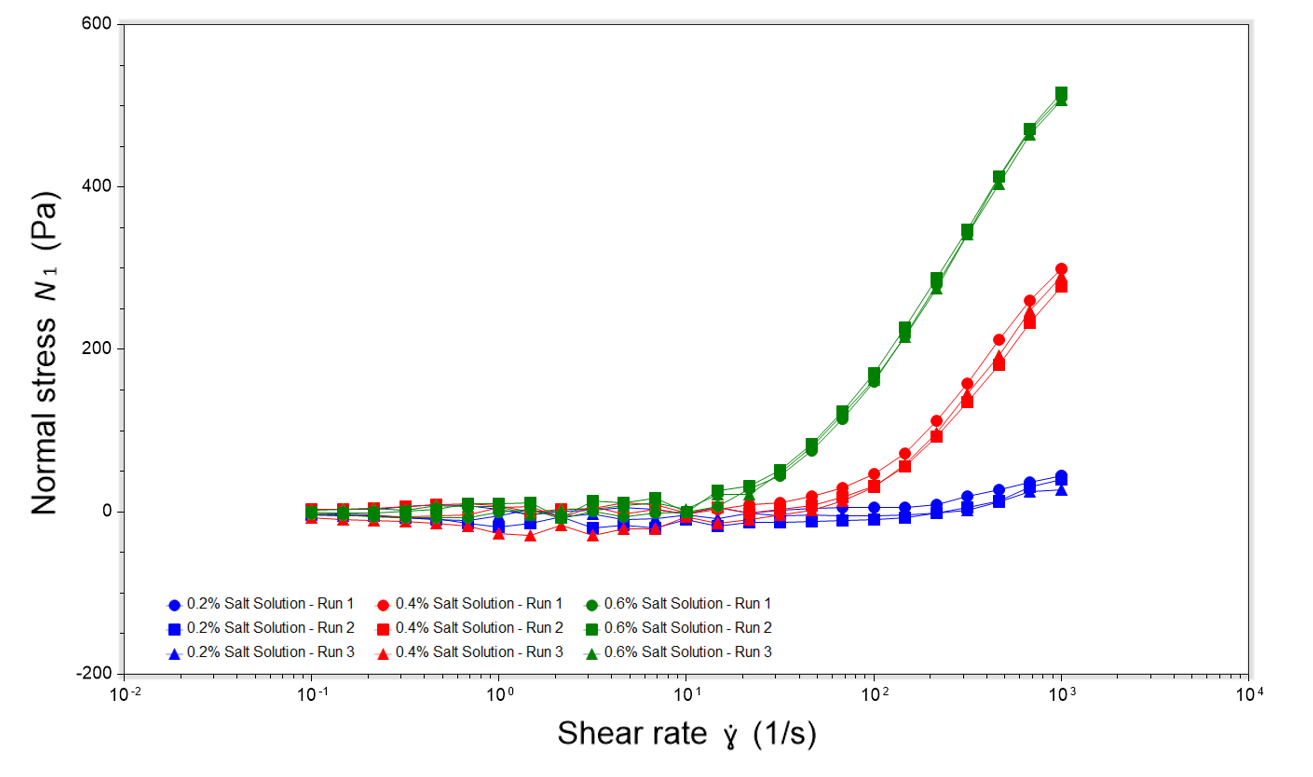
Normal stress measurements were taken for surfactant solutions for 0.2%, 0.4% and 0.6% salt concentration. For the surfactant solution containing 0.6% salt concentration, the normal stress rapidly increases at increased shear rates, showing the most pronounced increase out of all the samples. For the solution containing 0.4% salt concentration, the normal stress also increases at higher shear rates, with a less pronounced increase compared to the 0.6% salt concentration solution. The solution containing 0.2% salt concentration stays relatively constant across the range of shear rates, showing that this solution exhibits the lowest viscoelasticity, likely due to it having the lowest salt concentration.
Summary
The lab team at the Centre for Industrial rheology managed to carry out rapid testing to investigate the effect of salt addition to surfactant solution, taking a deeper look at the “salt curve” phenomenon. This case study showcased our advanced characterisation techniques and the depth of insights that our methods can uncover.
If you are interested in learning more about the rheology behind your formulations, do not hesitate to contact us.